A History Of The Atomic Model And The Experiments Behind It
Using a kitchen knife, cut a loaf of bread in two equally sized pieces. Then, cut the two pieces again to obtain four, and continue cutting the loaf into identical slices until you have the smallest bread slices in existence. In the abstract scenario that you were using an atom-wide kitchen knife, the result will have been atom-sized bread - which would be a rather wonderful experiment to carry out, I should imagine. This hypothetical scenario is what Greek philosophers Democritus and Leucippus proposed approximately 2500 years ago to explain matter; according to them, matter was composed of thousands of tiny, indivisible particles of different shapes, and they called these particles 'atomos' (which is Greek for 'uncuttable').
Nowadays, we know that Democritus and Leucippus were amazingly correct for their lack of scientific equipment, even if their ideas of atomos were purely hypothetical and could admittedly be somewhat abstract. (Supposedly, they claimed that iron atomos were shaped like hooks that allowed them to hold themselves together for strength and that salt atomos were spiky for their sharp taste. Novel, but not what we find.) Unfortunately, further work on the topic was discontinued in favour of the Aristotlian model for matter, which told of objects being made of differing values of the elements of fire, water, air, earth and ether - a concept popularised mainly due to Aristotle's fame as a philosopher. In fact, people accepted the theory for so long that scientists like Galileo only really began questioning it around the 1600s - more than two-thousand years after its proposal. Goes to show that you should never fully trust the scientist, even though they may be well-regarded - instead, always trust the data. (Note: this does not mean that you should ignore a professional's advice, but simply that it is wise to make your own research of the proof given to validate information, as it lets you make a more accurate judgement of it.)
It has since been analysed that objects like 'fire' are not made of single elements at all (which I actually discuss in my post on burning wood), and are instead atomic structures with varying energy levels that create the effects that we see naturally. In order to ascertain this, the British meteorologist John Dalton worked on the problem in the early 19th century, ultimately providing us with the first modern theory of the atomic structure.
Indivisible Particles & Plum Puddings
To corrobarate his hypothesis of what makes up matter, Dalton made experiments on a variety of compounds made from 8 different elements (which he wrote about on his book, 'A New System Of Chemical Philosophy') to show that chemical reactions formed products from the integer multiples of exact ratios of their constituent reactants. For instance, one of his most credited pieces of work involved reacting nitric oxide (NO) with oxygen gas (O2) under various conditions - namely, underwater and in sealed gas containers, with varying gas volumes added in different trials - leading him to realise that certain compounds could only be made from specific ratios of NO and O2 reacting together (e.g. dinitrogen trioxide, N2O3, would only be produced from nitrogen-oxygen masses in a ratio of 0.49:1). Consequently, Dalton concluded that different elements were all made of unique, indivisible particles - atoms - that were identical to atoms of the same element but were different to atoms in other elements, with oxygen atoms being heavier than nitrogen atoms.
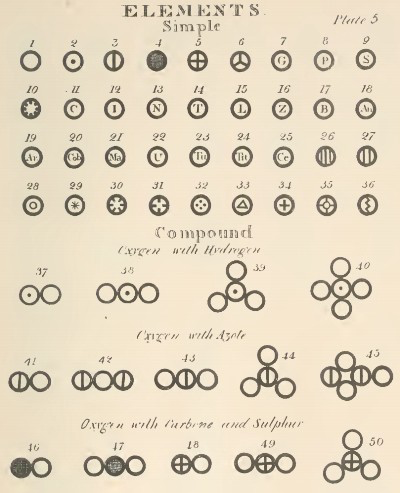
When John Dalton first published his findings to the public in 1808, the scientific community was generally rather noncommital to the idea that indivisible spheres - which Dalton called 'atoms' - existed in virtually every material possible in the universe. Many revolutionary ideas begin with much debate and controversy, however, so I think it quite fortunate that a select number of researchers nonetheless decided to entertain Dalton's ideas later on - eventually finding that he was, in fact, correct.
Dalton's model is now known as the Soild Sphere Model, and it made for a good starting point for further research on the structure of atomic particles, especially when finding their shape and composition (because Dalton's description of atoms as 'solid spheres' was never gonna cut it). This became the case around 100 years later, when Nobel Laureate Sir Joseph J. Thomson used some of the most advanced technology at the time to discover electrons in his famous cathode ray tube experiments. In principal, his procedure involved setting up a glass tube with a low internal gas pressure and running a high-voltage current in a metal sheet at one end of the tube, with a positively charged plate in front of the wire. As a result, this gave electrons in the sheet sufficient energy to be released from the metal surface (a phenomenon known as thermionic emission) and be attracted and accelerated towards the positive plate - until the electrons shot off and collided with the gas molecules in the cathode ray tube.
Without going into too much detail, these collisions energised the gas molecules enough so that they fluoresced and released light in return, which ultimately allowed Thomson to propose the existence of cathode rays - the particles that are now known as electrons. Thomson obtained the same result even after changing the material of the metal sheet and, by deflecting cathode rays in electric fields of known strengths, he was therefore able to show that cathode rays were both universally present and had mass. In other words, he proved that atoms were NOT indivsible and were actually made up of electrons.
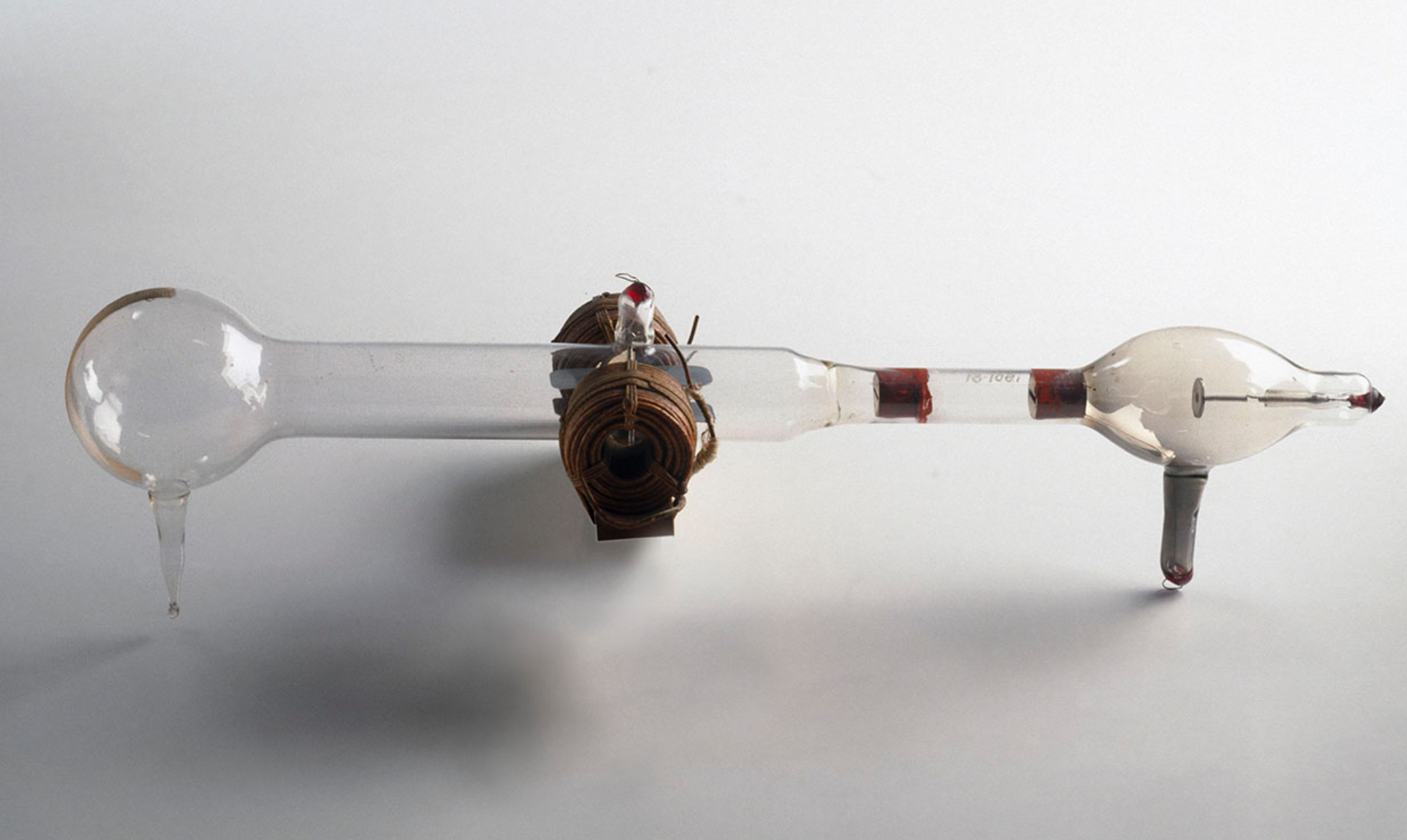
Thomson's atomic model, I should say, is probably my second favourite. Not because it is necessarily accurate - it isn't, really - but because it has what I think is a splendidly creative and colourful name. It is known as the Plum Pudding Model, because, according to Sir Thomson, the atom was shaped like a plum pudding - with electrons being the raisins and the rest being a moulded area of positive charge. Simply delicious. Encouraged by his discovery, nuclear physicists then started working on the problem more intensively - until, only 10 years later or so, a remarkably simple experiment resulted in the next landmark in particle physics.
Atoms As Tiny Saturns
When British physicist Ernest Rutherford first decided to purchase a sheet of pure gold for his experiment in 1908, I expect he was rather anxious that it would lead to some promising results - otherwise, it would probably have been a rather unfortunate event for his lab's funding. His reasoning for it was that gold, as a metal, is one of the most malleable materials in the market and could therefore be formed into the thinnest sheet of matter possible. Under his direction, Rutherford guided fellow nuclear researchers Hans Geiger and Ernest Marsden into firing a high-energy alpha-particle beam (a.k.a. positively charged radiation) into the gold foil, just to see what would happen (which, I find, is typically the thought process behind most interesting scientific revelations). This experiment is known as the Geiger-Marsden experiment - and it astounded Rutherford to no end.
Instead of bouncing back, as was expected, most of the protons actually went through the gold foil and spread to be detected by a radiation counter behind, with only a very small fraction of them returning the way they come from. Accordingly, Rutherford theorised that most of an atom was empty space, with all of its mass and charge concentrated in a centre that he termed the nucleus. Since the protons were deflected instead of being attracted into the nucleus, Rutherford further concluded that it had to have a positive charge.
Combining his results with Thomson's findings of the electron, Rutherford created a model of the atom that portrayed a single positive nucleus (with a volume about one thousandth that of the entire atom) being circulated by electrons, and it is known as the Nuclear Model. Very shortly thereafter, in around 1913, Danish physicist Niels Bohr expanded on Rutherford's ideas and used mathematical approaches and the observation of fluorescence in energised gas molecules to propose one of the first theories in quantum mechanics. In particular, he argued that atomic electrons did not get absorbed into the nucleus because they were fixed in position (or localised) inside discrete energy levels. You might have seen these as the Saturn-like rings in the popularised diagrams that depict the atomic structure - they are what Bohr referred to as orbits. Accordingly, this model was called the Planetary Model, and it was getting close to the real thing.
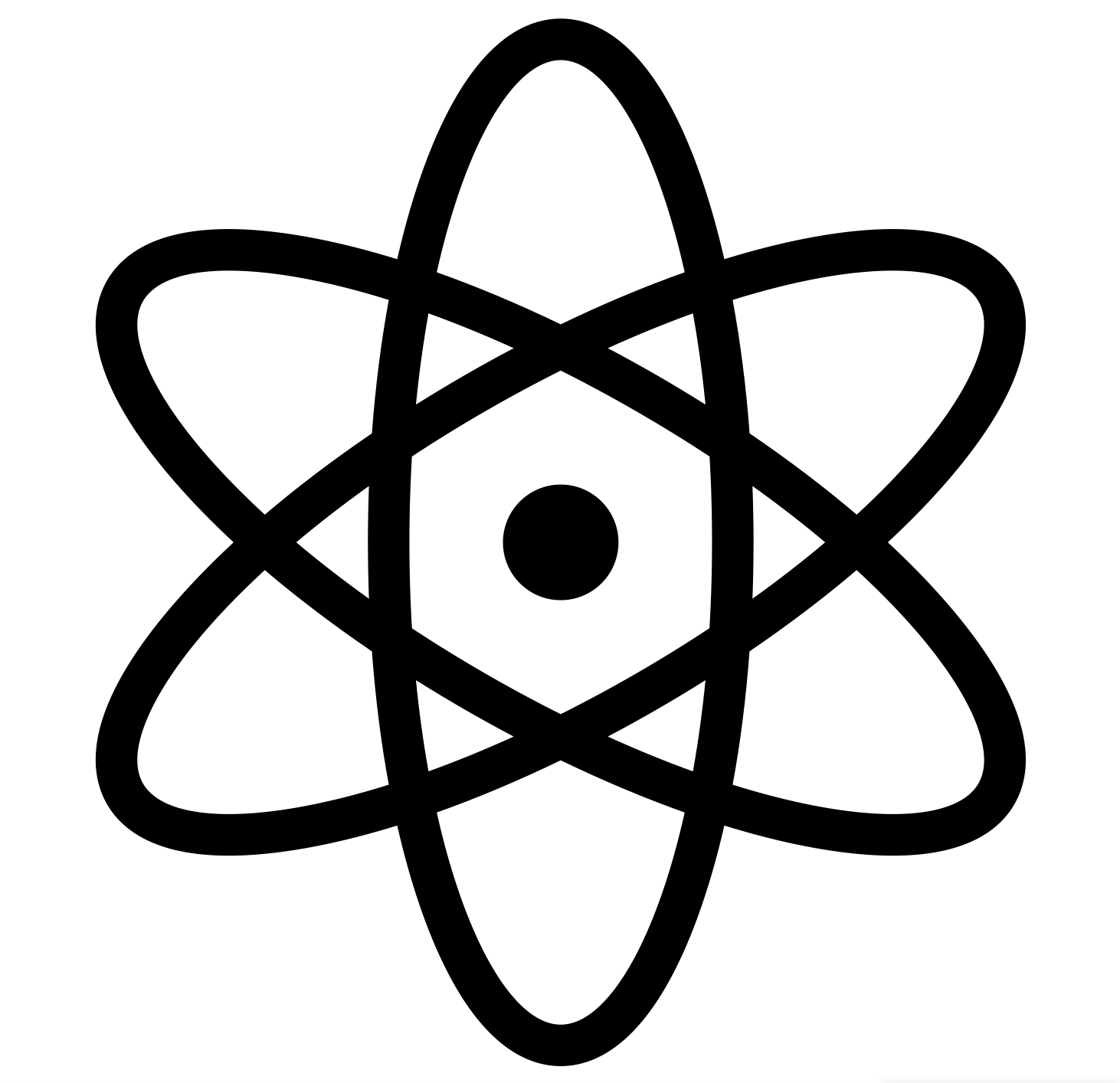
The Modern Model
Though it does serve a variety of basic purposes - like showing chemical bonds and introducing subatomic particles to high-schoolers - Bohr's depiction of the atom is somewhat simplistic. In reality, as Erwin Schrödinger found in 1926, electrons don't really follow set pathways in atoms. Instead, they jitter around all over the place (within the nucleus' vicinity), like excited mosquitoes constantly buzzing around right next to your face. Furthermore, quantum phenomena like particle-wave duality and superposition essentially allows them to exist as waves in multiple places in the atom at the same time - but we can generally still picture them as classical particles just fine.
Electrons do appear to almost always occupy 'preferred' volumes in space, however, and so we construed the concept of orbitals, which are really only 3-dimensional atomic orbits. Other work at the time also involved the discovery of protons, neutrons and the subatomic particles inside them (i.e. quarks), many of which were first detected in the Large Hadron Collider in Switzerland. All of this ultimately gave us the very accurate and rather complex model of the atom that we have today, usually known as the Quantum Model. Quite the progress from where we started, I think.
Albeit, this is not the end. As we advance in our understanding of quantum theories and mathematics, over time, our model will continue to be refined and revised to more accurately portray the atom. Just another thing to look forward to, I suppose!
References
- Berryman, S. (2016). Ancient Atomism. The Stanford Encyclopedia of Philosophy. Retrieved from https://plato.stanford.edu/entries/atomism-ancient/
- Usselman, M. C. & Leaist, D. G. & Watson, K. D. (2008). Dalton's disputed nitric oxide experiments and the origins of his atomic theory. Chemphyschem. Retrieved from https://pubmed.ncbi.nlm.nih.gov/18175369/
- Dalton, J. (1808). A new system of chemical philosophy. Cambridge University Press. Retrieved from https://doi.org/10.5479/sil.324338.39088000885681
- The Editors of Encyclopaedia (2020). Rutherford model. Encyclopedia Britannica. Retrieved from https://www.britannica.com/science/Rutherford-model
- Svidzinsky. A. A. & Scully, M. O. & Herschbach, D. R. (2005). Bohr's 1913 molecular model revisited. Proceedings of the National Academy of Sciences of the United States of America, 102(34), 11985–11988. Retrieved from https://www.ncbi.nlm.nih.gov/pmc/articles/PMC1186029/