Bioluminescent Fungi & A Light-Generating Metabolism
Truffles, in my opinion, are delicious. Though admittedly quite expensive, their mushrooms can be used to produce some of the most exquisite and unique tastes in pretty much all types of cuisine - particularly of the Italian kind. (Got to love truffle oil ravioli!) That said, I must admit that I am not as keen with other types of fungi. Most taste rather bland to me and, though I understand that most contain very healthy amounts of protein, I basically almost never eat them. My apologies to the fungus lovers out there.
I do find mushrooms quite interesting, though. They inhabit almost every country on Earth and have been around since the very beginnings of multicellular life, forming one of the oldest types of eukaryotic beings in present times. Like plants and animals, fungi make up their own sector of life - and they have an amazing amount of variation to show for it. Some are small, brown and unassuming, while others are bright red with white polka dots to tell you that eating them will result in you having an unfortunate time. Comparitively, some others are single-celled and live mostly isolated lives (e.g. yeast cells), with a certain few grouping and fusing together to form some of the largest single organisms on the planet (look up the Armarilla ostoyae for more details about this). In essence, however, all fungi have one basic purpose: to reproduce. Of course, this goes for pretty much all lifeforms that we know of, but fungi are an especially interesting case due to their practically unparalleled way of spreading offspring via their spores. This phenomenon is well-studied, and we have lists of characteristics that define different spores for different species, including how they are spread.
One of the ways in which fungi can do this is through bioluminescence (which is a recurring subject of study in biology, as shown by bioluminescent Hawaiian bobtail squids). From Asia and Europe to South and North America, most continents in the world feature mushrooms in their ecosystems - but only 71 of the over 100,000 fungal species have been identified to generate biological light. Though this makes them a rather obscure topic of study, people have been wondering about bioluminescent mushrooms for a while now - I understand that even Aristotle made observations of them back in the day 2500 years ago, referring to them as 'cold fire' in rotting wood - and so we do know a couple things about them. For example, all known species of bioluminescent fungi are known to emit low wavelength light, which typically makes them appear to us as shiny green mushrooms. Useful for parties, I suppose, but how could such a feature help them in the wild? Well, before we get into the 'whys' of their light-generating metabolism - how do mushrooms glow?
The Caffeic Acid Cycle & Generating Light
In order to glow, fungi use a series of chemical reactions within their metabolism that emit light as a by-product. Like most metabolic pathways in biology, these reactions can be fuelled by a variety of different other pathways, in turn producing other molecules that might be useful to the organism for a variety of different reasons. Given that these interchanges of biological molecules typically occurs between hundreds of pathways at a time, you can start to see how it might be difficult to strictly define a set pathway for how bioluminescence takes place. Albeit, we do have a general map for it.
First, we have luciferin, which is a fascinating type of organic molecule that produces light whenever it is oxidised (loses electrons) by a complementary enzyme known as luciferase. If you've ever wondered about the dazzling spectacle that is fireflies at night, it is also this interaction that generates light within them, albeit with a rather separate metabolic pathway that surrounds it. In the same way, there are different unique varieties of these molecules within different organisms, and, in regards to fungal species, their variant of luciferin is known as 3-hydroxyhispidin - though I will continue referring to it simply as fungal luciferin for the sake of clarity. Further, fungal luciferase has been labelled as Luz (which is a rather lovely name for it, I think, given that 'luz' means 'light' in Spanish).
Though development is still under way for the full description of fungal bioluminescence, one popular model made by a group of scientists proposes a self-sustaining cycle that produces fungal luciferin and thereby recycles it into a starting molecule after generating light. This starting molecule is caffeic acid, and it is one of the molecules that gives coffee its acidic properties. Of course, this coincidence is entirely unrelated to fungi, but it does pose the curious question of how related they are to plant species that synthesise the molecule naturally, such as the coffee bean family. Anyway. The cycle that follows - which is somewhat unofficially known as the caffeic acid cycle - occurs as described below:
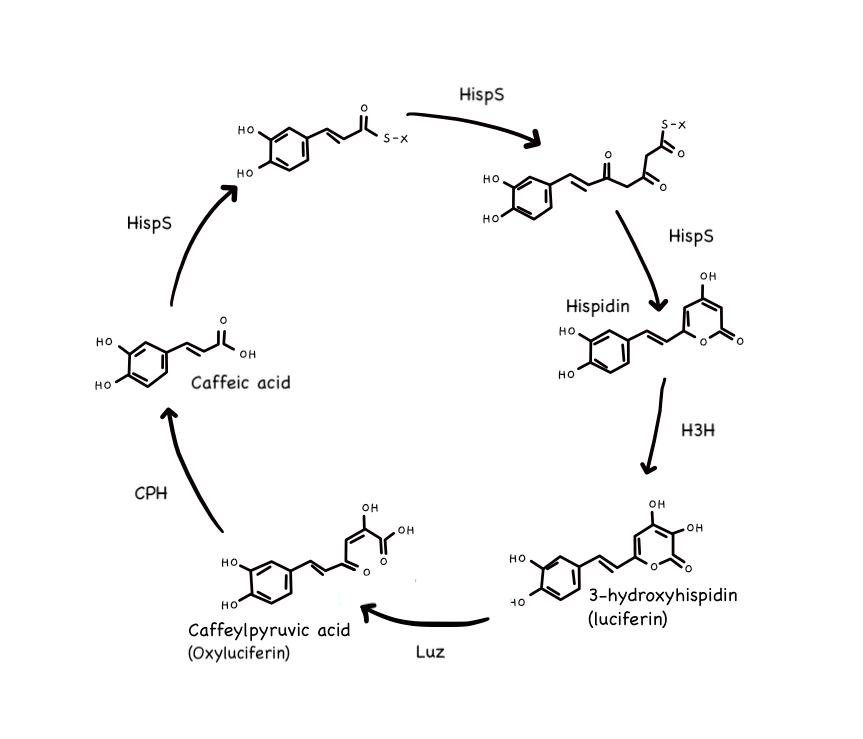
- First, caffeic acid is modified by hispidin synthase (HispS), an enzyme, to obtain a carbon ring and result in hispidin;
- Hispidin is then hydroxylated/hydrated with water by another enzyme called H3H, thereby turning it into 3-hydroxyhispidin, a.k.a. fungal luciferin;
- Quickly thereafter, Luz (the luciferase enzyme) switches the location of elctrons in fungal luciferin's added carbon ring by reacting it with an oxygen molecule, which creates a high energy (and very unstable) molecule that acts as an intermediate to the reaction;
- The intermediate molecule then spontaneously decomposes to caffeylpyruvic acid (otherwise known as oxyluciferin), producing carbon dioxide as a by-product and emitting the rest of its excess accumulated energy as green light;
- Finally, oxyluciferin is cut and turned back into caffeic acid by a catabolising enzyme called caffeylpyruvate hydrolase (CPH) - thereby starting the cycle all over again.
Though the pathway is admittedly a fair amount more involved and complex than this - with other energy-storing molecules reacting to fuel the metabolism of luciferin synthesis, for example - the shown steps are a good representation for how fungal bioluminescence is supposed to work. Nowadays, scientists use this model (albeit a much more sophisticated version of it, I would think) to more accurately predict the genes involved in producing bioluminescence and hence to try to transfer such properties to other organisms, such as plants. One particularly interesting experiment tested this idea by genetically engineering strains of Agrobacterium bacteria (which are cells specially adapted to essentially edit the genomes of complementary plant species with their own genomes) to include genes from the caffeic acid cycle, thereby growing the altered cultures next to tobacco plants to pass the genes unto them through lateral gene transfer. As tobacco plants naturally contain caffeic acid, the end result was clear: autonomously and ubiquitously glowing plants. The research behind this is incredible, and it would probably make for a great postgraduate research project for any enthusiastic biochemists out there (hint, hint)!
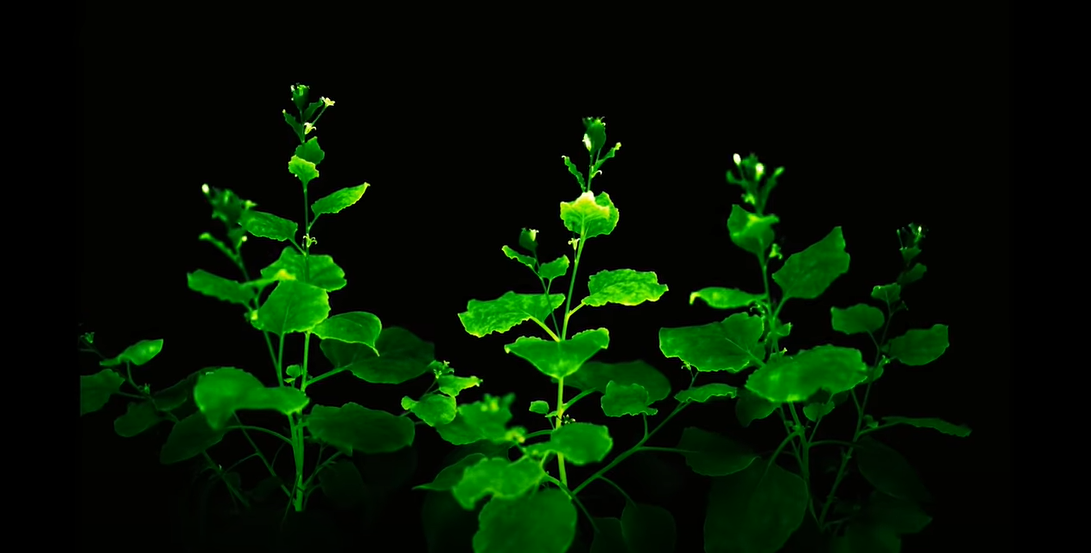
We now turn back to the original question, however: why do these fungi even glow in the first place?
Fungal Bug Lights
One study from 2015, led by Cassius Stevani at the São Paulo University in Brazil, obtained experimental evidence that flying insects were found more commonly at night around glowing mushrooms than their non-glowing variants. This was done by spreading fake plastic mushrooms carrying LEDs that imitated the light emitted by a native species of bioluminescent fungus (Neonothopanus gardneri) on the forest floor, thereby waiting to see the species of insects that would appear at the site of interest. Interestingly, the results showed a number of insects that are good at transporting fungal spores be attracted to the plastic mushrooms - even though they produced no identifiable scent or chemical signals that could cause this behaviour. In other words, the researchers found that bioluminescent fungi could use the light they emitted to spread their spores with the help of insects (which is a form of commensalism).
They also showed that such fungi use an internal circadian clock to be able to glow exclusively during night time - a mechanism similar to the biological day-night cycle of most animals, such as the one in humans or Monarch butterflies. We may therefore conclude that, contrary to the theory that fungal bioluminescence is simply a useless by-product of their metabolism, fungi actually glow as an adaptive response to their environment - a niche that allows them to more effectively spread by working together with their animal neighbours.
As with all kinds of scientific fields, the research for this phenomenon is still ongoing, and only now is it truly starting to shine (see what I did there?). Though humans have been able to genetically modify organisms for some time now, it is these kinds of obscure - perhaps even unorthodox - fields of study that allow us to come up with the most exciting technologies of the future. Before trying to insert fungal bioluminescence genes in plants, for example, scientists had tried to do it with bacterial genes - and, while they had achieved some light production, it had been dim and sporadic around the plant at best. Even in this front, progress is beinig made. And who knows? Someday soon, you may even find yourself switching your LED light bulbs to literal plant lights at home.
References
- Oliveira, A. G. et al (2015). Circadian Control Sheds Light on Fungal Bioluminescence. Current Biology, 25(7), 964–968. Retrieved from https://www.cell.com/current-biology/fulltext/S0960-9822(15)00160-8
- Kotlobay, A. A. et al (2018). Genetically encodable bioluminescent system from fungi. Proceedings of the National Academy of Sciences, 115 (50) 12728-12732. Retrieved from https://www.pnas.org/content/115/50/12728
- Reuter, D. N. & Stewart, C. N. & Lenaghan, S. C. (2020). Lighting the Way: Advances in Engineering Autoluminescent Plants. Trends in Plant Science, 25(12), 1176–1179. Retrieved from https://doi.org/10.1016/j.tplants.2020.08.004
- Mitiouchkina, T. et al (2020). Plants with genetically encoded autoluminescence. Nature Biotechnology, 38(8), 944–946. Retrieved from https://www.nature.com/articles/s41587-020-0500-9