Coding For Change - The Genetics Of Natural Selection
If there is one predominant theme underlying all of biochemistry, botany and zoology - indeed, all of biology - it would be statistics. The molecules that make us do not have a consciousness of their own, nor some intelligent purpose that drives them. Instead, particles simply follow the rules and properties that the universe has assigned them (e.g., what charge and mass they have, how much energy they carry, etc.) and flow down thermodynamic gradients to be as energetically stable as possible. As nothing is certain, this is governed by probabilities; even heat transferring from a hot to a cold object is not a fixed certainty, but simply an event that is very likely to happen. Heat transfer occurs from the movement of the trillion trillions of molecules that wonder around in space, however - so, when each one has a higher probability to be in one place than another, you can see why a warm cup of coffee doesn't usually grow hotter over time.
Concepts in thermodynamics and molecular statistics are applied time and time again in the life sciences, whether it is to explain why proteins fold the way they do or why an organism's fatty cell membrane can remain together while still retaining its fluidity. In a literal sense, it is also what allows information in our bodies to be encoded in the form of DNA, and could even be said to be responsible for the particular large-scale anatomy and behaviours of living animals. Although molecules had not yet been discovered, perhaps it is these kinds of ideas - simple probabilities repeating themselves millions of times, leading to a distinct direction for a biological organism's traits - that led Charles Darwin to propose his theory on natural selection in 1859. Indeed, I would argue that statistics is the very essence for evolution.
As Darwin was making his famous case study on the physical variation between the finches living on the Galapagos Islands, he made several observations on how their beaks might conjur advantagous characteristics for acquiring food; sharp, short beaks would allow insectivorous birds to grasp and capture insects more easily, while more rounded, larger bills would make it easier for other fruit and seed eaters to crush and bite on their feed. After some 30 years of going back and forward on ship to the Islands, and with close observations on the finches' similarities with those of birds on South American coasts, Darwin ultimately concluded that the variation had come from a differentiation of species over time from a past related ancestor. His book, 'On the Origin of Species,' spoke of such. The reason behind it was clear - organisms evolved to give them the best chance of survival in their environment, whether by optimising their fitness or altering their respective niche to reduce interspecific competition (i.e., competition for resources between birds of different species).
The exact mechanism behind how variation comes about in different organisms was uncertain at the time, but Darwin had a few ideas. In particular, he believed that small characteristics were acquired by organisms randomly over time, and that only the traits that proved beneficial to individual organisms got carried over through the generations. In his own words:
"This preservation of favorable variations and the rejection of injurious variations, I call natural selection or the survival of the fittest."
After much controversy since Darwinian theory was first established, scientists now widely agree that it is generally correct. Natural selection is very real, and it is what has brought the marvelous biological diversity that we see today. Darwin did not have modern apparatus for his experiments, however, and so probably did not realise the full extent of evolution - nor its implication in molecular statistics and even the tiniest of the machinery inside individual cells. So, what do we know?
A Gazillion Pathways Encourage Variation
We know that evolution, in its purest form, is all about genetics. It is in our DNA that information is stored and transferred, ecoding all the proteins that form a cell's function and role in the body. When specific sections of the genetic code change, it can therefore cascade and result in macroscopic, noticeable changes to the organism's phenotype. There are two overarching types of mechanisms that lead to genetic changes: mutations, occurring spontaneously in the entire body, and asymmetric cell division, which takes place in germline cells to produce gametes (a.k.a. sex cells) and is heritable to offspring. Each type has different consequences and scales in an organism's life, but germline cell divisions are the ones most commonly responsible for evolution, so we shall focus on them for now.
Another name for asymmetric cell division is meiosis. Although very similar to symmetric cell division (mitosis), which is how most of our body's somatic cells divide and allow our tissues to grow and repair themselves, meiosis in most organisms is strictly contained in the sex organs (like ovaries or testes). Typically, body cells contain multiple copies of DNA-encompassing chromosomes (homologous pairs in diploid cells) for quality control, and meiotic division takes advantage of this to produce a greater number of gametes per cycle of division. To go into some detail, meiosis proceeds as follows.
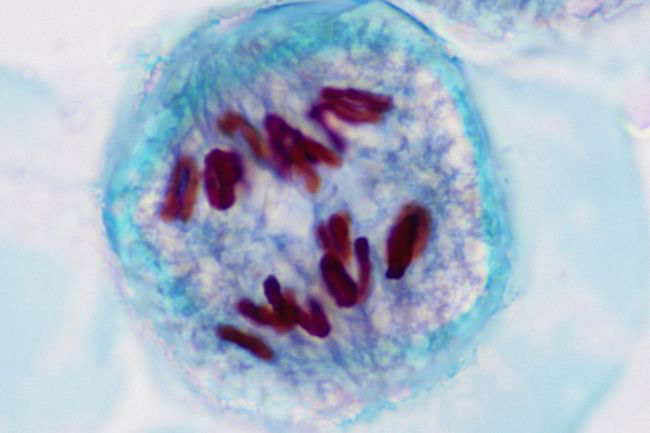
- A germ cell, which contains a full set of homologous chromosome pairs in its nucleus (23 pairs in humans), replicates and condenses its DNA to form visible individual chromosomes. The nuclear envelope encompassing the chromosomes further disintegrates to allow them to move. This stage is known as prophase.
- Afterwards, in metaphase, chromosomes are aligned with their partners along the equator of the cell. Here, protein filaments start to pull each homologue apart in a stage called anaphase.
- Finally, during telophase, the homologues reach their respective pole of the cell and become encircled by newly formed nuclei, at which point the cell splits its cytoplasm in two (cytokinesis) to ultimately produce two daughter cells. The entire cycle repeats itself once again, but this time pulling apart the two halves of individual chromosomes within anaphase to ultimately end up with four haploid gametes.
Around the time that the chromosome homologues come together in the first cycle's prophase, their proximity allows the crossing over of specific sections of DNA between the pair. While this process is admittedly far more complex and regulated than it sounds, it is in essence the most significant player in the creation of genetic variation between different chromosomes. You see, specific genes do not usually remain constant in biology, and instead change over in diverging species to create unique versions of themselves. Individual versions of a single gene are referred to as alleles, and these are what crossing over scrambles around in meiotic division.
If we exclude this exchange of alleles and only take into account the random segregation of chromosomes in the second round of meiosis, an average human with 23 chromosome pairs would be able to create a total of 8,324,608 possible combinations of gametes. When you don't ignore crossing over, then, you might start to realise just how much variety there is in our genome - as well as how amazingly unique you really are in the human population.
The journey doesn't end there, however. Only recently, molecular biologists have taken notice of the true significance of further processes controlling not just our genetic code, but also how much each gene is expressed in a cell. The study of how DNA expression can change without alterations in its basic code - which a lot of the time is still hereditary - is known as epigenetics, and it is at the forefront of pretty much all biochemistry research today. Epigenetic changes are far more liable to occur in one's lifetime than mutations, with both the environment and our lifestyle influencing how they develop in us. (Unhealthy habits and activities have the unfortunate tendency to have much impact in our epigenetics, with smoking, for example, increasing the expression of cancer-causing alleles.)
To summarise: our cells are really good at promoting genetic variation through cell division and a response system malleable to their environment, thereby introducing evolution in a species by the natural selection of favourable traits in their offspring. Now, while this is all well and good, it raises the question of just how worth it this incredibly energy-demanding process is. Clearly, genetic variation is very worth it; though bacteria do not display meiosis, even they have eomplex mechanisms that help shuffle genetic information between different cells of a population. What kind of advantages can variation truly bring, then?
Natural Selection In The Lab
Since Darwin's time, researchers have found the genes responsible for the varying morphologies of South American finches. One of these, which produces a protein labelled Bmp4, has been identified to be particularly relevant in birds with large, broad beaks, while it is mostly absent in sharp-beaked finches. As we have seen, such phenotypic variability is a construct of the selective pressures endowed by a species' environment - including their surrounding competition, hazards and accessibility to limited resources. In the case of Galapagos Islands finches, geographical separation of groups in an original population of the birds probably led to their speciation, which by disruptive selection created the biodiversity that we now see in them.
Interestingly, some evolutionary biologists have also proposed that variation and sexual reproduction decreases the amount of codependent genetics between individuals of a species, thereby reducing the effect of genetic drift (where interbreeding in a small population leads to rapid spread of an allele throughout the population over generations) and increasing the impact of natural selection. In other words, sex makes a species fitter and stronger in the world more quickly than if it was left to grow alone. No wonder!
Albeit, natural selection is not an exclusive privilege in sexually reproductive organisms. Indeed, even tumour cells (which divide by mitosis) have the nasty habit of encouraging mutations in their own DNA to change via directional selection to most easily and effectively survive in a host body. Cells grown in labs also showcase similar adaptive qualities (thought not as severely as cancer), making natural selection quite a pain when you wish to investigate isolated cells to see how they might work in their natural environment.
References
- Abzhanov, A., Protas, M., Grant, B. R., Grant, P. R., Tabin, C. J. (2004). Bmp4 and morphological variation of beaks in Darwin's finches. Science 305(5689):1462-5. Retrieved from https://doi.org/10.1126/science.1098095
- Andrews, C. A. (2010). Natural Selection, Genetic Drift, and Gene Flow Do Not Act in Isolation in Natural Populations. Nature Education Knowledge 3(10):5. Retrieved from https://www.nature.com/scitable/knowledge/library/natural-selection-genetic-drift-and-gene-flow-15186648/
- Crouch, D. J. M. (2017). Statistical aspects of evolution under natural selection, with implications for the advantage of sexual reproduction. Journal of Theoretical Biology. Retrieved from https://doi.org/10.1016/j.jtbi.2017.07.021
- Hughes, P., Marshall, D., Reid, Y., Parkes, H., Gelber, C. (2007). The costs of using unauthenticated, over-passaged cell lines: how much more data do we need? Biotechniques 43(5):575, 577–8. Retrieved from https://doi.org/10.2144/000112598