Exploring The Amorphous, Siliceous Solid That Is Glass
Glass, believe it or not, is one of the most complex materials we have observed on Earth. It is also incredibly useful for manufacturing windowed houses, glass furniture, a number of electronics, and a variety of other very practical and every-day objects that we probably take for granted. Humanity has recognised this potential since the Bronze age. Ever since the Sumerians found glassmaking around 4,000 years ago in the Mesopotamian civilisation, people all around the world have been implementing the material in their work consistently - though nowadays glass is more common than ever before. Recent advances in material science research have only further intensified interest in glass, and for good reason.
Being made from the molten construct of silicon dioxide, or silica - which constitutes most of the sand that we find on the beach - glass is unique in that, at room temperature, it takes a much different shape than the sand from which it originally came. Both are made of the same chemical elements, so why do their properties differ so much? Well, one reason is that glass is not actually only made of silicon dioxide - that is only a part of its overall constituents, forming the groundwork for the multilayered structure that is to come. And, even then, silicon dioxide does not only stay as silicon dioxide when it is heated into the molten liquid required to make glass. Instead, it experiences a structural change that not even diamond obtains when it is made from coal. Indeed, glass is definitely more complex than diamond - as we shall see in a moment below.
The Structure Of Sand & Glass
It might surprise you that most sand is technically considered a crystal by geologists. Literally, 'sand' is defined as a granular substance resulting from the erosion of siliceous rocks - which are mineral formations made up mainly of silicon dioxide, such as quartz or granite - and does not offer a strict chemical composition for it. However, since quartz is one of the most commonly appearing minerals on the planet surface, it is generally thought to be the main component in the sand that we use to make glass. As such, when I use the word 'sand' in this post, please be aware that I am referring to grains that are made exclusively of plain quartz - i.e. of only silicon dioxide.
In its solid state, silicon dioxide (SiO2) exists as a large molecular structure with strong chemical bonds binding it together, known as a covalent lattice. The SiO2 molecules comprising this lattice are in a tetrahedral geometry - which looks similar to the shape of a square pyramid. Since all molecules in the lattice are locked into the same formation, with regularly spaced identical units throughout, scientists refer to it as being crystallised. This is also why I previously mentioned diamond; like silica, it is made of tetrahedral molecules locked in a covalent lattice and is also a crystal, though with carbon atoms instead of silicon and oxygen. Because of this, both materials have a great many properties in common - such as a high chemical stability. In regards to quartz/sand, its molecular structure makes it an incredible strong and stable material. Add this to the strength of the covalent bonds (defined as the direct overlap of electron orbitals) between the atoms, and you get things like a melting point of roughly 1,700ºC - which is what we observe in real life.
When people do manage to get it to a high enough temperature, however, the structure falls apart. So much so, in fact, that it can become a different material altogether - glass. In comparison to the crystalline structure of silica in sand, the elements in glass are more randomly ordered and more susceptible to fractures, hence why it is so brittle. I have also seen a couple of people spreading around that glass is actually a liquid, albeit a very viscous one. This is wrong. Instead, glass belongs in a subcategory of solids known as amorphous solids, which include plastics, rubber and asphalt (all three of which, I might add, are carbon-based!). At room temperature, all of these materials are solid, meaning that the molecules that constitute them are not able to move around each other. This is because the covalent bonds holding molecules together are simply too strong to allow it, although such can change again at suffieciently high temperatures. Naturally, such amphous structures are not as strong as their respective lattices, with different types of glass having melting points of down to 600ºC (much lower than the one of sand). Finally, do note that modern glassmaking usually involves adding further chemicals to produce composite glass, done precisely to give it desired qualities not present in regular glass (e.g. when a mixture of metal oxides are added to make toughened glass).
Now, why in the world are these chemicals so rare? And, if their formation is so random to begin with, why are amorphous solids not actually liquids (at room temperature)? To put it simply: elemental silicon and carbon just have some of the most useful chemistry there is.
Silicon As An Element
Only very few chemical elements are able to form amorphous solids. Due to their ability to form four stable covalent bonds with other atoms, carbon and silicon are two of them; most others are either too rigid and cannot stably imitate the random nature of amophous structures, or too unstable to exist long enough for any significant solid formation. Carbon, especially, is particularly flexible to the formation of molecular structures (as we see in, you know, all living organisms on the planet).
Comparatively, scientists have only found a small number of silicon-based molecules - but those they have found are evidently incredibly useful to us. Besides glass, for example, an amorphous solid made entirely of elemental silicon - literally called amorphous silicon - is regularly used as a thin-film semiconductor by green-energy companies to make solar panels (ever used a solar-powered calculator, anyone?). This is because electrons in amorphous silicon are able to move around enough that a current can be created, with the added feature that they only move in one direction through the material. Furthermore, it may also be used to make a number of electronic components (such as LCDs) and even x-ray imagers. Pretty cool.
So silicon has some rather interesting properties that we can put to use. To improve on that and to optimise our handling of silicon, current research is focused on trying to more accurately visualise differing silicon-based structures, thereby allowing us to more easily predict how they will function. Strangely, however, it is actually quite challenging to do this - and so brilliant scientists all over the world are giving it a shot.
Visualising The Atoms In Glass
Just a few months ago (at the time of writing), a group of researchers led by Yao Yang from the Science & Technology Center in the University of California published a paper on the atomic structure of amorphous solids - metallic glass in particular. Beforehand, accurately imaging glass had been an ongoing issue in the scientific community, mainly for two reasons. Firstly, it's irregular structure posed a problem. For the past ten years or so, scientists had been using an imaging technique called atomic-resolution electron tomography to build up 3D images of atomic structures. This entailed directing a beam of high-energy electrons at a static structure and obtaining a 2D image from their refraction, rotating the structure periodically to get a selection of images that could be added together to produce a 3D mapping of it. As long as the material has a sufficiently organised pattern, or if it can at least be copied to study different orientations of it over time, we can build an image of it. Glass, however, is neither. It is simply too random and too variable between panes to get a universal depiction of it. So Yang et al had to try something new.
Instead of manually capturing the 2D images needed to construct their mapping, the team operated sophisticated computational algorithms to obtain a series of shots, which showed slight variations in contrast to when the glass was viewed in differing orientations. They further minimised noise and corrected distortions that might blur the images, and finally calculated averages of their findings to produce an accurate 3D model of metallic glass.
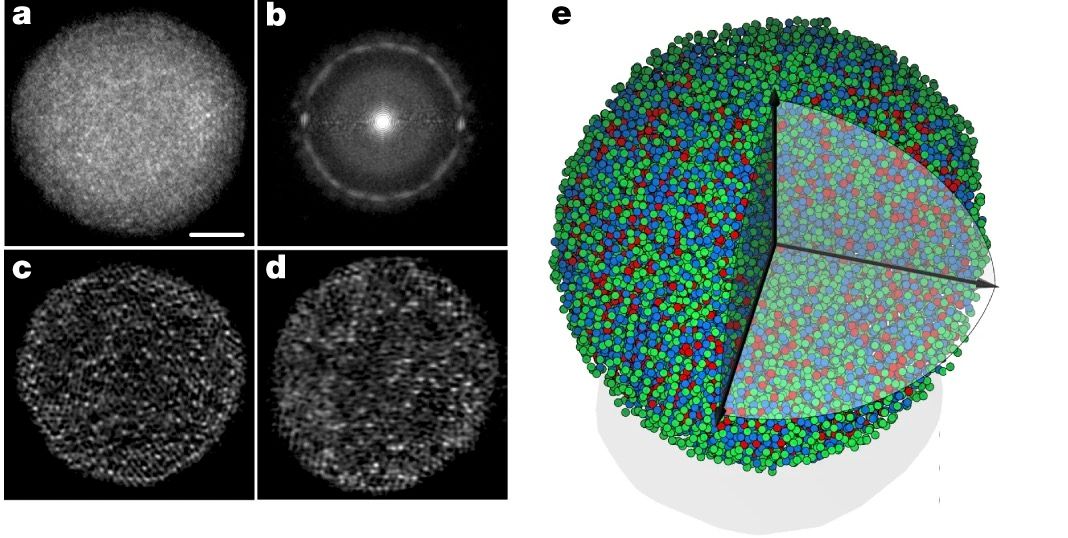
Even with these astounding findings, there is still progress to be made in visualising the atomic layout of glass. The team's model does not distinguish between individual elements, after all, only giving you an idea of the ones that they could be. This is also only a type of metallic glass that constitutes 8 elements; enough to be impressive, for sure, but still just an example of all the different kinds of glass that we have created as a civilisation.
But I digress. Modern technological advances have certainly made us a lot more aware of the chemistry behind amorphous solids, and I expect it will only continue to improve in the future. What will we be able to do with such findings? Perhaps we might improve our solar panel designs, or construct more efficient optical cables - or even just make the coolest windows in human history! We shall see. Now that you have read a bit about the complexity of your sand-based amorphous windows, though, take a moment to ponder about them. Neat, huh?
References
- National Center for Biotechnology Information (2021). PubChem Compound Summary for CID 24261, Silicon dioxide. Retrieved from https://pubchem.ncbi.nlm.nih.gov/compound/Silicon-dioxide.
- Masai, H. & Nishibe, T. & Yamamoto, S. & Niizuma, T. & Kitamura, N. & Akai, T. & Ohkubo, T. & Yoshida, M. (2021). Low melting oxide glasses prepared at a melt temperature of 500 °C. Scientific reports 11(1):214. Retrieved from https://doi.org/10.1038/s41598-020-80424-9
- McMillan, P. F. (2021). Machine learning reveals the complexity of dense amorphous silicon. Nature 589:22-23. Retrieved from https://doi.org/10.1038/d41586-020-03574-w
- Yang, Y. & Zhou, J. & Zhu, F. et al (2021). Determining the three-dimensional atomic structure of an amorphous solid. Nature 592:60–64. Retrieved from https://doi.org/10.1038/s41586-021-03354-0