Tumours In Trees - How Agrobacteria Genetically Modify Plants
If you go out to walk often, particularly in forested areas, you may have noticed a few trees with large bulks of mass growing from their trunks before. Demorphed and decrepid, these protuberances happen rarely in large dicotyledonous plants like oak trees, and they are neither intended nor adaptive features in the wild (at least from the perspective of the trees!). Nevertheless, they happen often enough that we give them a name - we call them crown galls. Galls have been witnessed to spawn in over 90 plant families, but they are most common in fruit trees (like apple, raspberry or almond trees), willows, maples, and even woody rose bushes and grapevines.
In essence, crown galls occur due to the uncontrolled and excessive division of plants cells in the body of a plant, and are therefore infamously regarded as a type of 'plant cancer'. This is not as severe it sounds, however, as crown galls do not have the capability of spreading throughout the organism as tumours do in us, with plant cells being too immobile (from the sticky extracellular matrix surrounding them) and close together to allow it. As such, if you ever find a somewhat unsightly growth appearing in your backyard tree, have no fear - your tree will not die soon. At most, it will probably suffer a somewhat stunted growth and take longer in producing fruit from a shortage of nutrients. Albeit, I would suggest that you still try to remove the lump as soon as possible, for crown gall disease is perennial and sticks around for the plant's life, growing steadily and almost unendingly as the tree becomes larger. (If you spot one, do as the Romans and take it down!) This is also why galls are most conspicuous on trees - their bulk is proportional to their host's size.
Plant tumours do offer an interesting opportunity for research, however. Given their relatively benign behaviour in plant species and their innocuous attitude towards animals (more on this in a bit), it is easy to experiment on them. Scientists know this - and their research has brought on incredible interest from agricultural industries. So, what exactly is it about crown galls that has them so enthused?
Attacking A Wounded Tree
Though crown gall disease does share its similarities with cancer, the ways that they spring up is generelly quite different from each other. Whereas in cancer cells typically become tumorigenic by obtaining random mutations that are overlooked by the cells' internal quality control mechanisms (though a few types to come from pathogens), galls are comparitively not very random at all; indeed, they are normally very much an intended product of their environment. Introducing - the bacterial family Agrobacterium. These aerobic cylindrical bacteria usually live in the rhizosphere of plant roots (which I wrote about in detail in my post about soil), trading nutrients with other species in their microenvironment to survive. However, whether it is due to bark-eating insects or human activity in grafting trees, occasionally their inhabiting plant will become vulnerable and exposed - and Agrobacteria will jump on the opportunity and take it by storm.
Like the human skin, plants are usually surrounded by a layer of dead cells that protect their live, functioning cells inside from exposure to external hazards like disease. This layer is known as the sclerenchyma, and it contains some of the strongest (waterproof) lignin cell walls in the organism. When it is damaged, it leaves room for bacteria to interact with the plant's softer layers, known as the collenchyma and parenchyma - live photosynthetic tissues that have roles in acquiring, storing and transporting food around the body. In other words, a treasure haul for the voracious bacteria outside. Of course, even though it can vary in strength from cell to cell, all plant cells (including the ones in softer tissue) are surrounded by a cell wall. Usually, this is enough to resist any foreign invasion of pathogens long enough for the sclerenchyma to grow again, and so not every wound on a tree will give it crown galls. Alas, should the wound be large and unmanageable enough, Agrobacteria will be able to attack - and they do this through the multi-step process of lateral gene transfer.
Making A Factory
The main culprit behind this is the bacterial species Agrobaterium tumefaciens. This species contains large transmembrane protein receptors (so they pass through and are attached to its cell membrane) that bind to molecules that occur in larger quantities when a tree has been wounded, including the phenolics and sugars dissolved in tree sap. This binding then triggers a signalling cascade within the bacterial cell that induces certain genes in its DNA to be displaced and processed into single-stranded transfer DNA (ssT-DNA) - not to be confused with RNA, which is also single-stranded - that associates with a VirD2 protein at one end to be led towards the cell's membrane. At this point, the bacterium in question will be placed adjecent to a plant cell, forming a pore complex that leads from the bacterium to the plant cell's cytoplasm (sort of like a miniature syringe that penetrates the plant cell). Through this pore, the ssT-DNA is led into the plant cell and associates with a set of VirE2 proteins (also transported through the pore) that allows it to enter the cell's nucleus and move closer to its own DNA.
Here, things get a bit muddled up. Overall, researchers have found that the ssT-DNA/Vir protein complex is somehow able to split apart and leave only the T-DNA strand free to join the plant's genome, thereby introducing foreign instructions into it that are favourable to the A. tumefaciens bacterium. However, the details of how this can occur are actually yet to be fully understood. Some models suggest that the ssT-DNA binds directly to a strand in an unwound plant DNA double helix - while other, more recent models propose its conversion into a double helix first, followed by its integration into a break in the plant DNA. Either way, the plant's genome ends up with an involuntary extension from a parasitic organism - hence the name lateral gene transfer - which changes its metabolism.
The bacterial genes inserted into the plant DNA have two purposes. First, they code for ezymes that metabolise sugars and amino acids into molecules called opines, which are thereby secreted out of the cell for the surrounding Agrobacteria to absorb them for sustenance. At the same time, other inserted genes code for a different variety of enzymes - referred to as phytohormone synthases - that quickly begin to synthesise plant hormones normally used by the plant to grow via cell division. As these enzymes start to continuously be produced in larger amounts, the cell starts to receive more and more hormone signals that make it divide consistently and uncontrollably - leading to a crown gall. Furthermore, all cells produced from this division will be identical, and so they too will feature opine-creating and dividing properties. The cycle goes on, and on, and on...although it fortunately slows down once the gall has become large enough to stop receiving sufficient nutrients for continued growth.
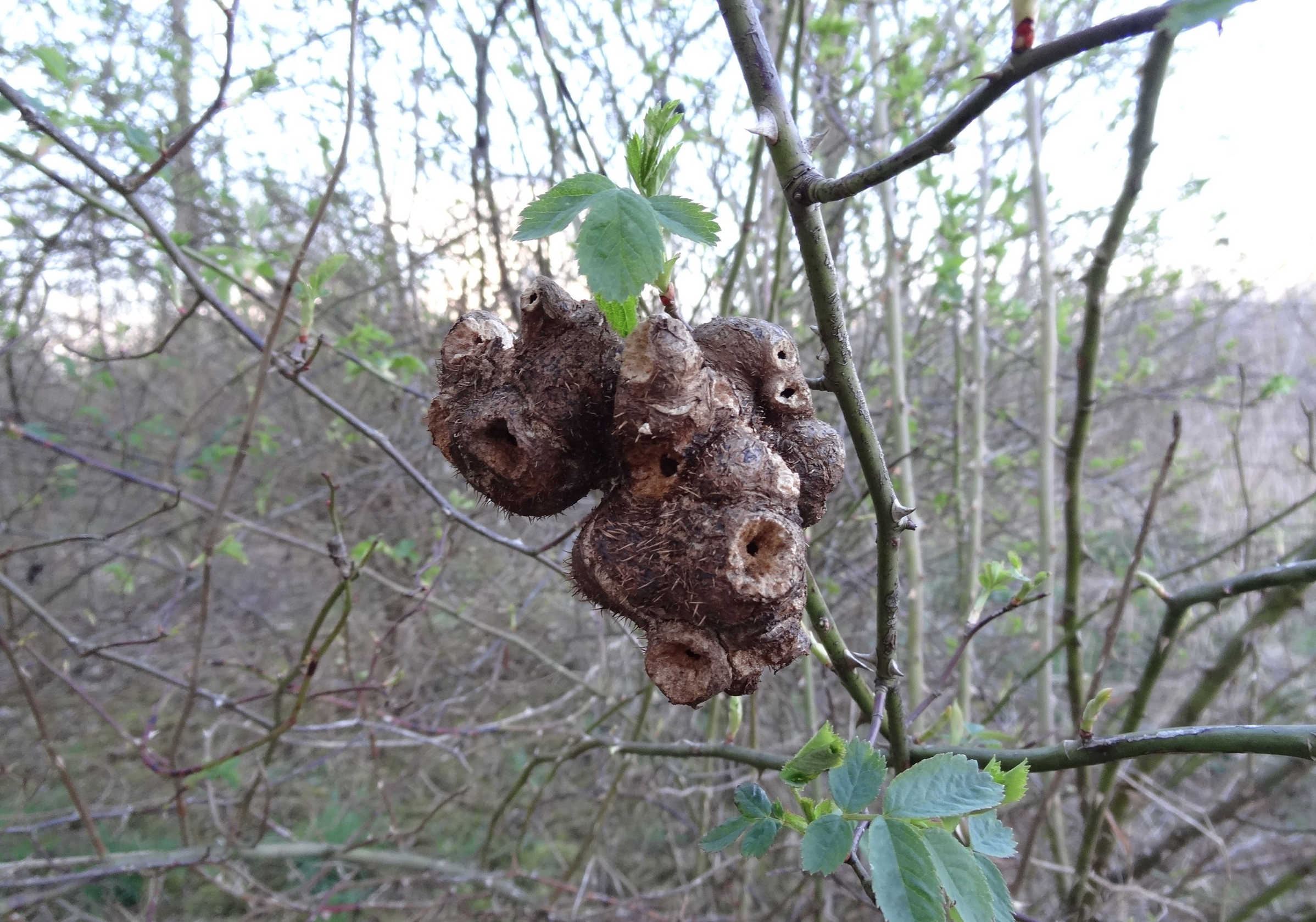
But this is interesting. Can you see what has happened here? These unicellular organisms - small, fragile and comparitively simple to the plant cells as they are - have essentially managed to turn the tree's cells into a self-assembling and autonomous food factory for themselves. Not only that, they have done so through genetic engineering. For us, a crown gall might be an unsightly form of a natural tumour. And yet, for A. tumefaciens, it is actually a field of genetically modified crops that it has tended to unequivocally by itself. In other words, humanity was never the first species to tamper with genetic modification; it was bacteria.
Modifying Bacteria To Modify Crops
Fortunately for us, bacteria are not adapted to cause uncontrolled proliferation in animals like us, and so you will most likely never find someone get cancer directly from them. As far as I can tell, their targets lie pretty much exclusively with plants, and scientists have spotted natural remnants of their behaviour even in our own farms. One study made by an international collaboration of universities and scientific research institutions - including the International Potato Center, in Peru, which I am simply delighted exists - even tested 291 samples of cultivated sweet potatoes grown from Asia, Oceania, Africa and South and Central America, and found that every single one contained traces of Agrobacterium T-DNA. Of course, this does not mean that 291 sweet potato farms are overgrown with crown galls, but simply indicates that events of lateral gene transfer have been occuring far into the past.
Taking inspiration from this, agricultural scientists have tried genetically modifying plants directly by themselves - and it didn't work. Or, at least, it did not work very well. As such, they instead attempted altering the genome of the much simpler and easier to predict A. tumefaciens bacteria - and now we are using them routinely to add the exact genes we want into our crops. Examples of crops genetically modified like this include Roundup Ready soybeans, which are resistant to the herbicides that Monsanto sells, and virus-resistant papaya in Hawaii.
Now, I should mention that GM crops are not inherently toxic or dangerous or anything like that (as a lot of people might suggest). Disease-resistant crops can stop entire orchards from being wiped out (from ring virus in the case of papaya plants), and many modified crops can further contain nutrients desperately needed by nutritionally deficient societies (e.g. golden rice providing vitamin A to places with high blindness rates, like south-east Asia). Certainly, it is crucial that people handle this type of biotechnology responsibly, but I think they hold promise.
And, again, GM crops are natural - they have been produced by bacteria for millions of years now, after all.
References
- Doonan, J. & Sablowski, R. (2010). Walls around tumours — why plants do not develop cancer. Nature Reviews Cancer 10, 794–802. Retrieved from https://www.nature.com/articles/nrc2942?foxtrotcallback=true
- Zhu, J. et al (2000). The bases of crown gall tumorigenesis. Journal of Bacteriology. Retrieved from https://pubmed.ncbi.nlm.nih.gov/10869063/
- Liang, Z. & Tzfira, T. (2013). In vivo formation of double-stranded T-DNA molecules by T-strand priming. Nature Communications 4, 2253. Retrieved from https://www.nature.com/articles/ncomms3253
- Kyndt, T. et al (2015). The genome of cultivated sweet potato contains Agrobacterium T-DNAs with expressed genes: An example of a naturally transgenic food crop. PNAS 112 (18), 5844-584. Retrieved from https://www.pnas.org/content/112/18/5844
- Ream, W. (2009). Genetically engineered plants: greener than you think. Microbial biotechnology, 2(4), 401–405. Retrieved from https://www.ncbi.nlm.nih.gov/pmc/articles/PMC3815900/