Nuclear Bombs - A Powerful Science Used For Devastation
I've been thinking about nukes recently, likely as a result of the terrible humanitarian catastrophe taking place in Ukraine at the time of writing. In this way, I am also probably not alone. Knowing that a missile could obliterate your home and country at any time - and do worse - is a dreadful, depressing feeling for practically everyone aware of the news on Earth. And, while I do not pretend to know what it is truly like to live in Ukraine right now, such can only be doubly as true for the many unfortunate civilians at the front of the war. Nonetheless, such is the time we live in. As unlikely as many claim it to be, the threat of nuclear bombings is very much a possibility - though I do hope that it stays that way forever, without ever actually becoming something more.
I should point out that nuclear science is not all about explosions. In the most stripped sense of the matter, this field of study really just concerns itself with the presence of and thermodynamics behind atoms and subatomic particles. What is the energy change when uranium atoms are split into two? What happens if you crash two protons into each other while they're moving almost at the speed of light? Do atoms actually look like plum-puddings? As inconsequential as these questions might seem, the answers to them is what has allowed (and is allowing!) physicists to map out both the smallest and largest of objects in the universe. Nuclear physics involves more than just explosions. It is more than just deadly weaponry. Yet knowledge is a powerful thing; and, just as it has the capability of bringing us wealth and peace, it can also cause destruction and war.
Before we take a look at the weapons themselves, it is important to understand what laws of nature make them function in the first place. As such, we come across the basics of what holds all matter together: nuclear energy.
Nuclear Forces
Most probably, you will already have covered radioactivity at some level of education before. Here, we build upon our knowledge of the structure of the atom - that it is made of protons and neutrons in the centre (collectively called the nucleus), with electrons orbiting it in space - to show how higher energy states can lead to it emitting energy away in the form of radiation. This energy can take on a wide variety of forms depending on the conditions of the atom, transforming its particle constituents to release as much energy as possible. If there are too many protons in the nucleus, for example, one of them might be converted into a neutron, electron and antineutrino in a process known as ß-emission to decrease the electrostatic repulsion inside the nucleus (with the electron and antineutrino acting as the excess energy emitted away). Similarly, neutrons can be turned into protons when the reaction is favourable - though admittedly in a manner not quite the opposite of the above.
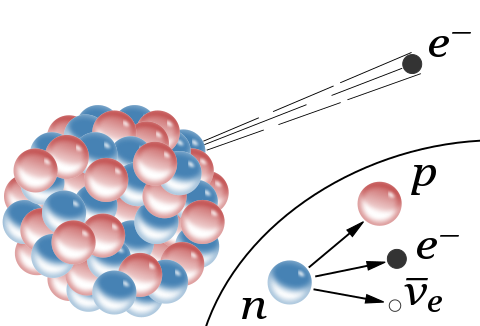
Theoretically, all particles are able to be converted into each other if the right combinations are crashed together. In simple terms, this is because all matter and antimatter in the universe is just a condensed form of energy, which can sometimes take the form of light, protons, electrons, and so on. While the details behind this phenomenon are far more complex than described, what we can take from this idea is that the energy of one particle is not fixed and is very much transmutable (again under the right conditions). This very fact has been observed experimentally before. In practice, it might surprise you that protons do not have the same energy when they are outside an atom as when they're a part of it. I do not mean that they are vibrating differently, either - their very mass is actually lower when inside the nucleus. We can prove this by adding together the masses of individual free nuclides (protons or neutrons, also called nucleons) to get a prediction for the total mass of an element's nucleus, and then comparing the result with the value observed experimentally. Given that the observed mass is always smaller than predicted, physicists refer to this effect as the mass defect.
When the mass defect was discovered in 1905 - by none other than the legendary Albert Einstein himself - you can imagine how surprised scientists would have been at the revelation. The particles they had thought were constants of all matter, after all, were not constant at all. Indeed, it is now commonly believed that nuclides must release some of their energy before they can form stable nuclei, lowering the nuclides' potential energy to bind them together. For this reason - and since mass and energy are properties of each other - the mass defect is sometimes also referred to as the nuclear binding energy. We can then use the equation ΔE=Δmc² (where ΔE is the binding energy, Δm is the mass defect and c is the speed of light) to convert between the two. As stable nuclei contain less energy than the same number of free nuclides making them up, nuclear binding energies are also always expressed as negative values.
You may notice that there is an interesting dilemma here. If protons and neutrons are at a lower potential energy when inside the nucles, indicating higher stability, what force is allowing it to happen? It cannot be the electromagnetic force, as protons are positively charged and would repel each other, preventing them from coming close together. Moreover, as this electrostatic repulsion does not disappear within the nucleus, the force responsible would actually need to be greater than it at short distances for a nucleus to form. We can therefore rule out gravity, which is generally far weaker than electromagnetism (though both are prominent players on planet Earth). Fortunately, however, there are two more candidate forces that have since been discovered by physicists: the weak nuclear force and the strong nuclear force.
Together with electromagnetism and gravity, the two nuclear forces make up the four fundamental forces of the universe. As the names imply, one force is also stronger than the other, with each typically operating in different nuclear reactions. The weak nuclear force, for instance, has been found to drive radioactive decay in unstable atoms (as described above) via W and Z boson vector intermediates. Comparatively, the strong nuclear force acts through gluon intermediates and essentially 'glues' quarks together when they are about 0.7-2.5 femtometers apart (where 1 femtometer = 1 millionth billionth of a meter). Crucially, since some quarks have the same charge and repel each other by electrostatic repulsion, this means that the strong nuclear force is greater than its electromagnetic cousin (at least at short distances). Being made of quarks themselves, protons and neutrons are both products of this attraction - and it is this that lets them join together in the nucleus.
Now, do note that there are a few strange intricacies with the strong force. You see, though it is definitely very strong, it is also (as I like to think of it) very picky. While it will hold nuclides fairly close together, the strong nuclear force stops being so cuddly once the distance between them shortens to below 0.7fm. At this point, it instead causes the nuclides to fiercely repel with a magnitude that is larger than even the electrostatic repulsion between protons at the same distance. Stupefyingly, the opposite then becomes true when the nuclides grow further apart, with the force's strength fading to almost nothing after 2.5fm. Like a parasitic bird, it keeps its hosts neatly in line with each other, positioning them at the most energetically favourable position when possible or otherwise ejecting them outside the atom.
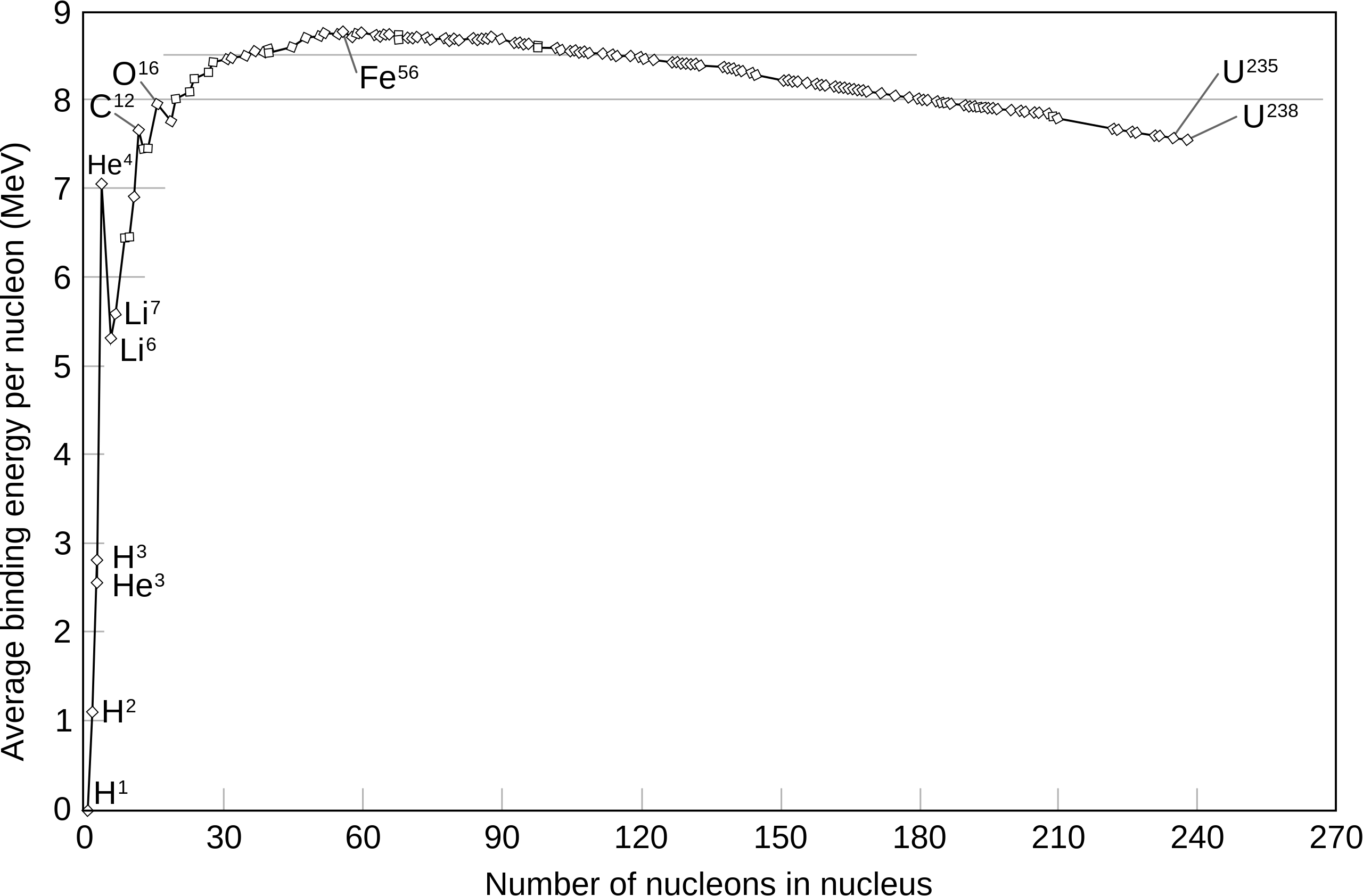
The behaviour of the strong nuclear force means that there is an optimum for nuclear composition, wherein nuclides are at just the precise numbers and distances apart to reach the lowest potential energy possible. In practice, this optimum is seen in the nucleus of iron-56, which is the most stable element known to us. We can measure nuclear stability by the binding energy of the element (as shown by the graph below); the higher the binding energy, the more energy is emitted when forming the nucleus and the more stable the involved nuclides are. Interestingly, this further tells us that all elements turn into iron-56 when given enough time. Eventually, smaller atoms like hydrogen will fuse, while larger ones like uranium will decay into lighter elements. The results, in turn, are the two principles behind the unfortunate weaponry at our disposal: nuclear fission and nuclear fusion.
Nuclear Weaponry
Nuclear fission is the process by which unstable atoms split into fragments, and it is most common in large elements like uranium and plutonium (which feature low nuclear binding energies). When this occurs, neutrons are sometimes emitted as a waste product of the reaction. Should these neutrons proceed to collide with other atoms in the vicinity, the transferred kinetic energy can cause them to also become unstable and split - ultimately producing a highly exothermic and explosive chain reaction.
Discovered in 1938 by German physicists Otto Hahn and Fritz Strassman, nuclear fission was first applied to war when the United States launched the top-secret Manhattan Project after Pearl Harbor in 1941. Through the guidance of J. Robert Oppenheimer (a man widely known as the 'father of the atomic bomb'), the original prototype of nuclear missiles was built. In this case, a tough metal casing was filled in three separate sections, one with an explosive propellant and the other two containing half of the critical mass of uranium needed for uncontrolled fission to begin. Once the bomb was a thrown, the idea was to propel the two uranium masses together and have them react, creating more and more pressure until the casing burst open and chaos ensued (hence labelling it the gun-type bomb). Destructive enough as this was, however, it was only the beginning of the project.
In the following years, continued work on nuclear bombs found plutonium to be a more effective catalyst for explosive fission, leading to the creation of plutionium bombs. In comparison with the previous gun-type model, these had a spherical mass of plutionium surrounded by shape charges, explosive propellants that blasted in one direction. When the charges went off, the implosion generated forced the plutonium to pack together tightly enough to reach critical mass - creating the devastation seen in Nagasaki in 1945 and killing approximately 50,000 people in the process. (The bomb in Hiroshima was similar and killed about 80,000, though it contained uranium due to a shortage of extracted plutonium.) No other nuclear bombs have been used in armed conflict to date.
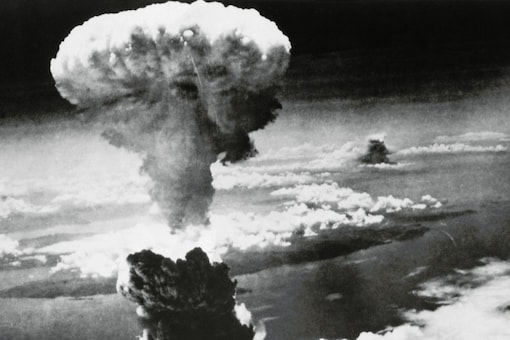
Frighteningly, the bombs from 1945 - each producing over 15 kilotons of energy (equivalent to fifteen thousand tonnes of TNT) - pale in comparison to the ones we have today. Only seven years after the bomb in Hiroshima, the first thermonuclear bomb (or hydrogen bomb) was tested in Eniwetok Atoll, a coral atoll in the middle of the Pacific Ocean. Encased with uranium-238, a small fission bomb is made to explode next to fusion material (made primarily of lithium deuteride) while styrofoam absorbs the gamma radiation emitted in order to heat and compress it. Finally, neutrons released in the reaction allow the lithium and deuterium ions to fuse, immediately splitting thereafter to form helium and tritium. With this, humanity had successfully made a 10 megaton bomb in 1952 - exuding over 670 times the destruction of the first plutonium bombs. Since then, Russia has tested a 67 hydrogen bomb, and nine countries in the world each owns tens of the things. Overall, the science behind them is definitely interesting - but I think it would be a good idea to keep it theoretical. Forever.
References
- Anon (2022). Nuclear Binding Energy. Openstax CNX. Retrieved from https://phys.libretexts.org/@go/page/454
- Vasiliev, B. V. (2015). About Nature of Nuclear Forces. Journal of Modern Physics 6(5):648-659. Retrieved from http://dx.doi.org/10.4236/jmp.2015.65071
- Sidel, V. W. & Levy, B. S. (2008). Weapons of Mass Destruction. International Encyclopedia of Public Health. Retrieved from https://www.sciencedirect.com/topics/social-sciences/nuclear-weapons