Sugar: A Sweet Poison Or A Chemically Diverse Nutrient?
As a matter of fact, sugars are not inherently bad. It is a common misconception that featuring sugar in your diet has all sorts of ill consequences - higher risks of obesity, heart disease and diabetes being the most widely known - but this idea is simply not true. Not completely true, anyway. Everything in excess has its own associated problems, whether it is drinking too much coffee, working to exhaustion or eating too many chocolate bites. In the same vein, however, even sugars are healthy (and even essential!) in moderation. Fruits like bananas or watermelons (or even tomatoes and cucumbers) are incredibly rich sources of sugar, and we are certainly not dissuaded from eating them.
When we look further into it, we further realise that sugars and carbohydrates are, for all intents and purposes, one and the same. To put it into perspective, the term 'carbohydrates' only refers to organic compounds (which are comprised of a carbon backbone) that contain oxygen and hydrogen atoms in a 2:1 ratio. Chemically, this means that the general formula for all carbohydrates is this: Cx(H2O)y. With this in mind, we can see how individual carbs and sugars only really differ by their length and arrangement of atoms in space. Admittedly, this does sound fairly insubstantive. Nonetheless, the difference is great enough that pretty much all forms of organisms use some kind of sugar to live. The question arises, then - what determines the role and function of separate carbohydrates?
Sugary Building Blocks
Similar to proteins (also known as polypeptides), carbohydrates are made of single monomer units joined together to form a longer polymer. Like building blocks, the orientation and number of these monomers determines the exact properties of their constituent polymer, ranging from its solubility and melting point to how it is broken down by an organism. Trouble is, there is quite a list of different monomers out there - and not all of them are natural.
All monosaccharides (the term for sugar monomers) are categorised and named based on the number of carbon atoms present in the longest carbon chain of the molecule. To picture this, imagine drawing a cross where the horizontal line is longer than the vertical one; if we take the lines to be representative estimates of the number of carbon atoms in a molecule, then the name of the molecule would be designated according to the length of the horizontal line only. Inspired by the constancy and simplicity of numbers, biologists hence give different monosaccharides their own Greek numerical prefixes. A diose is a two-carbon chain, trioses are three-carbon chains, tetroses are four-carbon chains, and so on. If you were really keen, you could probably even continue this into the double digits (though I would be surprised if their were many monomer sugars of that size).
Interestingly, the vast majority of monosaccharides actually belong to the group of hexoses. In particular, dietary sugars - glucose, fructose and galactose - are all hexoses with similar chemical compositions, and so people generally don't pay attention to the rest of their folk. Nonetheless, there are many other monosaccharides that play important roles in the proper function of cellular metabolism, some of which have been shown to be essential for life in general. Deoxyribose and ribose sugars, for instance, are pentoses that make up the bulk backbone of genetic material in the form of DNA and RNA strands respectively.
Additionally, a tetrose known as erythrose is furtively recognised to have wide-ranging responsibilities in ATP production (which is how cells store energy) and metabolite transformation in cells. Recent studies have further demonstrated that erythrose phosphate may even be oxidised into compounds (such as 4-phosphoerythronate, or 4PE) that display inhibitory mechanisms with metabolic enzymes in both eukaryotes and bacteria, the latter of which seems to be particularly susceptible to 4PE. With continued studies, immunologists could very well find sugar derivates that may be used as antibiotics in the future.
With that said, it is true that most pathways involving sugar can be traced back to the aforementioned trio - the trifecta of sugars, even. Combinations of these monosaccharides can produce other sugars, such as the disaccharides sucrose (glucose and fructose bonded together) or lactose (glucose and galactose). As such, it is only appropriate that we take a closer look at the chemistry and impact behind them, and so we shall do so down below.
The Molecular Organisation Of Sugars
Depending on the orientation of atoms in space, certain molecules can have completely different characteristics than molecules otherwise identical in composition. This typically happens when carbon atoms are bonded to four different groups around them. Since covalent structures like this have restricted levels of rotation due to the electrostatic repulsion between electrons, it means that the groups in this case are essentially 'locked' in place - unless sufficient energy is used to interconvert them. (If you are curious, I have also written a different blog post that further expands on the topic of the structure of the atom.) Such chemicals are referred to as stereoisomers.
Naturally, stereoisomerism is especially relevant when studying biochemistry, in which most chemicals you deal with are organic. As sugars are no exception, these, too, display stereoisomerism - though they do so in a way that is oddly unique to them. If you have studied biology before, you might already be familiar with the structure of glucose as being a cyclic hexose ring with a hydroxymethyl group (CH2OH) at the top. This is how glucose commonly exists in nature, and it is usually drawn in what is called the Howarth projection. Albeit, when considering the differences between aplha- and beta-glucose (yes, sorry for the surprise, but there are actually multiple forms of each monosaccharide), it is useful to consider the Fischer projection of the molecule. This form is more theoretical, and it is drawn somewhat like a stick-figure, as shown below.
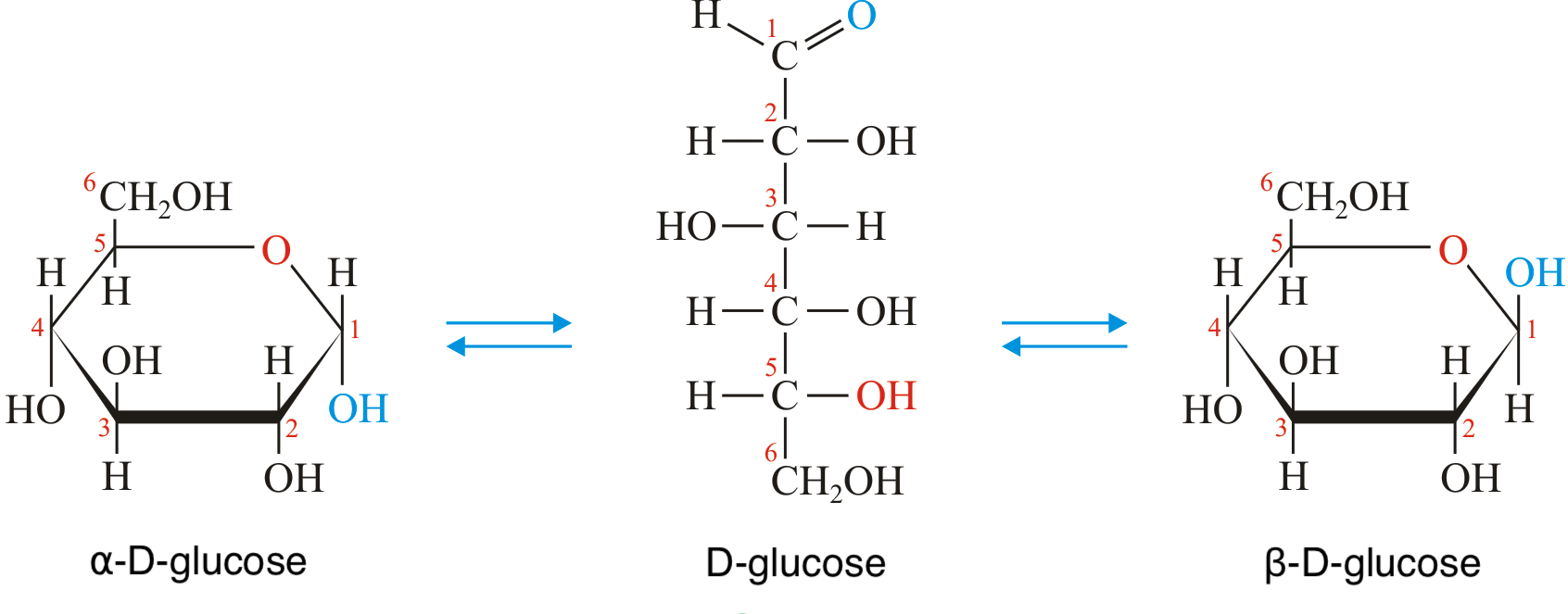
Without delving into too much detail of the organic chemistry at play here, note that the only difference between the two Howarth projections is the relative position of the hydroxyl (OH) group on carbon-1. This arises from how the aldehyde (CHO) group in the Fischer projection is oriented as the cyclic ring forms, further telling us that these variations - anomers, as we call them - only take place after cyclization. (Also, if you are wondering where the letter 'D' comes from, it is simply a label that distinguishes the above form of glucose in yet another type of stereoisomerism. D-glucose, to be specific, is the non-superimposable mirror image of L-glucose. These are known as enantiomers - though only D-glucose has been found to occur naturally in living organisms.)
Now, you might be thinking, why is this important? Surely, such a minor change in configuration could not possess sufficiently meaningful consequences to warrant so much concern over it. If glucose were to always exist alone, that might be right - but it doesn't. In most creatures, glucose is stored as long polymers of itself to act as either an insoluble energy storage or for support. Cellulose, for example, is a beta-glucose polysaccharide found around plant cells that provides strength and support to the cell. Due to the orientation of the hydroxyl group that we looked at, the beta-glucose units are joined by glycosidic bonds that force them to 'flip-flop' in organisation, so that each unit is upside-down relative to its neighbours. In turn, this arrangement makes the polysaccharide fibrous and linear, allowing several cellulose molecules to pack tightly together and withstand both osmotic and mechanical pressures in the surroundings (though they are still rather liable to combust).
Comparatively, starches like glycogen or amylopectin (which serve as energy storages in animal and plant cells respectively) are composed entirely of alpha-glucose subunits that all bond to each other upright. Not only does this permit side branches to form from the main saccharide chain - as alpha-glucose monomers can form glycosidic bonds with two other molecules - it also prevents polymer molecules from getting overly close together. Ultimately, this means that enzymes and other molecules can easily approach the chains and interact with them, rapidly and efficiently providing the cell with energy or a place to store excess sugar. Since these are fibrous molecules, they are also very insoluble in water, meaning that they can be formed without disrupting the water potential in the cell (or act as reservoirs in case the cell needs to change it).
Of course, glucose is not the only monosaccharide that can form different polymers. Fructan is a polysaccharide of fructose, usually existing in thistles and grasses to confer storage and resistance to the cold. Similarly, galactose can polymerise to form galactan, another polysaccharide important in the structure and composition of plant cell walls - particularly when young. Fact: pandas are quite keen to eat young bamboo shoots as a result of their high concentrations of galactan and hemicellulose (a shorter form of cellulose).
Saccharine Research
Besides investigating the eating tendencies in pandas (unironically a very important field of study), researchers are also attempting to see if they can modify sugars to treat a variety of medical conditions. Already, radioactive fluorine-enriched glucose is used by hospitals to check where it is absorbed the most in a patient's body, thereby allowing them to identify the location of a tumour via a PET scan.
Alternative forms of sugar could also be useful for people with conditions like Irritable Bowel Syndrome or heart disease. Typically, the processed sugar that we are told is bad for us is simply purified sucrose (which I mentioned above). It is actually also found almost universally in fruits and plant-based products like honey, acting as an alternative energy source in cells for when glucose is lacking. The difference, however, is that fruits contain a whole variety of sugars, vitamins, minerals, antioxidants, a bunch of water and more. To put it simply, the nutrients balance each other out, making fruits net healthy for us (though consuming too many bananas in one day will still cause high blood sugar).
Similarly, organic honey is a mixture of sugars and other nutrients that could be healthy in reasonable quantities. Recently, researchers from the Universiti Malaysia Kelantan, Malaysia, even postulated that honey produced by stingless bees could be used to tackle diabetes, as it is high in trehalulose - a different form of sucrose that is digested more slowly in the gut. Another pro for keeping beehive communities intact, and some lovely news for the people. Healthy sugar, anyone?
References
- Prinz, P. (2009). The role of dietary sugars in health: molecular composition or just calories? European Journal of Clinical Nutrition 73:1216–1223. Retrieved from https://doi.org/10.1038/s41430-019-0407-z
- Sachla, A. J. & Helmann, J.D. (2019). A bacterial checkpoint protein for ribosome assembly moonlights as an essential metabolite-proofreading enzyme. Nature Communications 10:1526. Retrieved from https://doi.org/10.1038/s41467-019-09508-z
- Tabachnikov, O. & Shoham, Y. (2012). Functional characterization of the galactan utilization system of Geobacillus stearothermophilus. The Febs Journal. Retrieved from https://doi.org/10.1111/febs.12089
- Rao, P. V., et al (2016). Biological and therapeutic effects of honey produced by honey bees and stingless bees: a comparative review. Revista Brasileira de Farmacognosia 26:657–664. Retrieved from https://doi.org/10.1016/j.bjp.2016.01.012